Qubits and bits with Clément Javerzac
In 2022, the FHNW School of Life Sciences made the bold move to establish a research group focused on applied quantum computing, becoming the first University of Applied Sciences in Switzerland to enter the quantum space. Led by Clément Javerzac, the group is on a mission to apply quantum computing to life sciences.
Prof. Dr. Javerzac, tell me what’s happening at the FHNW School of Life Sciences in quantum computing.
We’re starting with education: making students and faculty quantum aware. We held a first course in the Master's in Medical Informatics in 2023, which gave students the courage to tackle what can seem like a complex technology. In 2024, a quantum computing module was included in both BSc and MSc programmes, and we held a summer school at the FHNW on this topic.
You don’t need to be a mathematician or a physicist to try quantum computing. There are user-friendly tools and packages like the IBM Quantum Composer that can help you get started. What you do need is an understanding of which types of problems quantum computing is good at solving.
How do you identify the right research questions to apply quantum to?
Working alongside life science researchers provides an excellent opportunity for interdisciplinary exchange. I ask people to bring me their problems! But I don’t guarantee that quantum can solve them all.
One application we are working on is simulating nuclear magnetic resonance (NMR) spectra that are too complex for classical supercomputers. Quantum algorithms can be used to decode the composition of matter for new materials, for example to identify the best structure for a material that can capture CO2.
Quantum sensing is more mature and promising for medical applications, for example, diagnosing functionally relevant coronary artery disease (fCAD). The heart’s tiny magnetic field can be measured with quantum sensors. Together with Joris Pascal from the Institute for Medical Engineering and Medical Informatics, we are developing a magneto-cardiograph (MCG) as a point-of-care device powered by quantum sensors, in collaboration with CSEM and the University Hospital Basel.
What types of problems should quantum computing stay out of?
In contrast to AI, quantum computing is not good at solving problems that involve big data, because it is slow to load it. Quantum is ideal for solving combinatorial problems where there are few variables in the input, and many possibilities in the output. Think supply chain and logistics, scheduling shift work or DNA sequencing. With hybrid algorithms, you can get the best of both worlds: you can ask AI to solve a part of the problem (very fast), and quantum the other (very hard).
Which quantum computers is the FHNW School of Life Sciences currently accessing?
We book computation time on the best infrastructures in the world, including IBM’s quantum computers in the United States. This privileged access is made possible through our partnership with QuantumBasel.
We’re also excited about building European capacity in quantum computing. IonQ is establishing a European quantum data centre in collaboration with QuantumBasel, consisting of two systems with 35 and 64 algorithmic qubits respectively. This will position the Basel region as one of the most powerful quantum computing centres in the world.
Classical computers used to take up entire rooms and be used by specialists. Now they fit in our pocket. Do you see a similar trajectory for quantum computers?
I don’t think quantum computers will replace current computers, because different types of processing units have different functions.
- Central processing units (CPUs) make up the core of today’s laptops and mobile devices.
- Graphic processing units (GPUs) first appeared for graphic design and video game applications, and are now used to train deep learning models.
- Tensor processing units (TPUs) and data processing units (DPUs) are the next generation of processors designed specifically for big data applications and centres.
- Quantum processing units (QPUs) are best for solving hard, combinatorial problems without replacing classical computing.
Before quantum computers can make it to prime time, we need to increase their size: not only the number and quality of qubits, but the number of operations they can do, which is called circuit depth. The National Center of Competence in Research: Spin Qubits in Silicon (NCCR-SPIN) is working on alternative wafer-scale quantum circuits that can be fabricated by the semiconductor industry. Scaling up from qubits in the double digits to the millions will bring us into a new era of quantum computing.
What does the future of quantum computing look like?
We’re moving away from quantum supremacy toward quantum utility – understanding where quantum can make a practical difference. At our HackLife events, companies bring us their problems and we look at a variety of approaches, including AI and quantum, to see how we can solve them.
As the next generation of applied quantum experts graduate from the FHNW and other organisations, they will move to industry and become ambassadors of the technology. I’m excited to see where we stand in five years, with this new cohort applying the technology to drug discovery, materials science and medical devices.
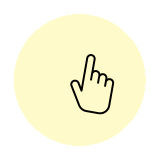
Key facts | |
---|---|
Partners: | uptownBasel, QuantumBasel, CSEM, University Hospital Basel, Creative Destruction Lab (CDL), NCCR Spin, Swiss Quantum Initiative. |
Financing: | SNSF 51NF40-180604, Innosuisse, NCCR-Spin, SCNAT. |